Neutrinos, often referred to as the “ghost particles” of the universe, hold the key to some of the most profound mysteries in astrophysics and particle physics. These elusive particles are produced in massive quantities during cosmic events such as supernovae, nuclear reactions, and gamma-ray bursts. Despite their abundance, neutrinos are notoriously difficult to detect because they rarely interact with other forms of matter. However, advances in neutrino detection technology have opened new pathways to understanding the universe’s most enigmatic phenomena. This article explores how neutrino detectors work, their impact on scientific discoveries, and what secrets they continue to unveil about the cosmos.
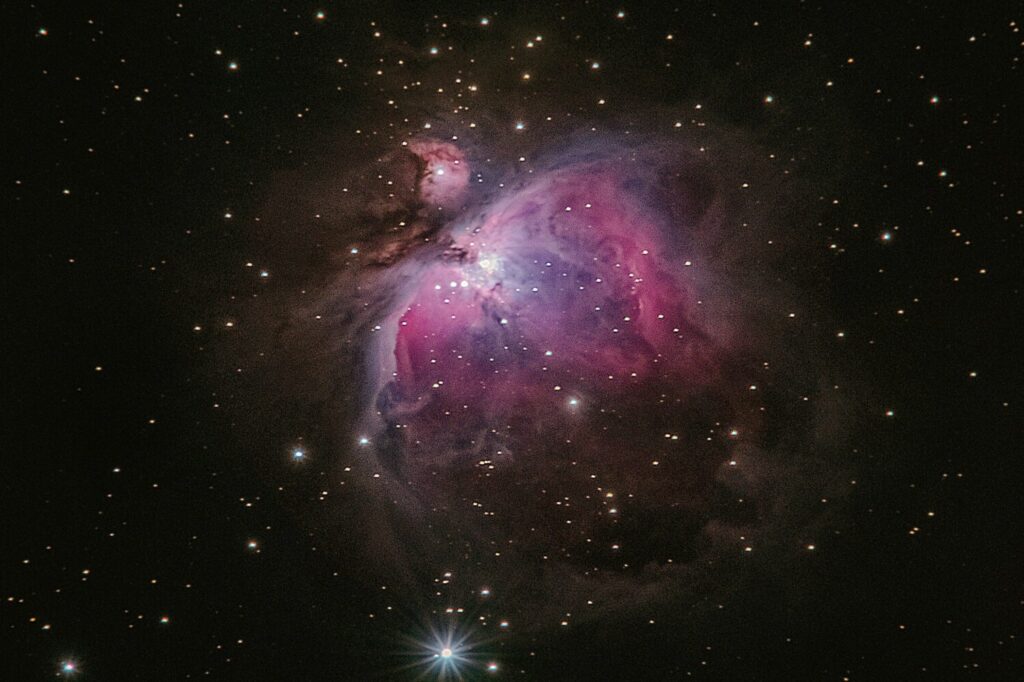
Understanding Neutrinos
Neutrinos are subatomic particles with no electric charge and a minuscule mass, making them some of the smallest and least understood components of the universe. Discovered in 1956 by Clyde Cowan and Frederick Reines, neutrinos were first proposed decades earlier as a theoretical solution to a puzzle in beta decay. They belong to the family of leptons and come in three known types, or “flavors”: electron neutrinos, muon neutrinos, and tau neutrinos.
Neutrinos are produced by a variety of natural and artificial processes, including:
- Stellar processes: Inside the Sun, nuclear fusion generates billions of neutrinos every second, most of which pass through Earth unnoticed.
- Explosive events: Supernovae release an incredible number of neutrinos during the collapse of massive stars.
- Atmospheric interactions: Cosmic rays striking Earth’s atmosphere result in neutrino production.
- Human-made sources: Nuclear reactors and particle accelerators also emit neutrinos.
Despite their prevalence, detecting neutrinos is a monumental challenge, requiring highly sophisticated instruments and deep underground facilities designed to minimize background noise from cosmic radiation and other sources.
How Neutrino Detectors Work
Neutrino detectors are specialized devices designed to capture and study the interactions of neutrinos with other particles. These interactions are rare because neutrinos primarily pass through matter without any measurable effect. To overcome this difficulty, researchers employ various detection methods based on the environments most conducive to capturing these fleeting events.
Common Neutrino Detection Methods
- Cherenkov Radiation Neutrinos occasionally collide with atomic nuclei or electrons, producing charged particles that travel faster than light in a medium like water or ice. This creates a faint bluish light known as Cherenkov radiation, which detectors can capture and analyze.
- Scintillation Scintillators are materials that emit light when charged particles pass through them. Neutrino detectors use these materials to amplify the signals from neutrino interactions.
- Radio Wave Detection Some advanced detectors, such as those in Antarctica, utilize radio waves to capture neutrinos interacting with ice or other dense materials. These methods are particularly effective for high-energy neutrinos originating from deep space.
Major Neutrino Detectors
Modern neutrino observatories are feats of engineering that push the boundaries of human knowledge. Some prominent facilities include:
- Super-Kamiokande (Japan): A water Cherenkov detector located deep underground that studies solar and atmospheric neutrinos.
- IceCube Neutrino Observatory (Antarctica): A detector embedded in the Antarctic ice sheet, specializing in high-energy neutrino detection.
- Sudbury Neutrino Observatory (Canada): A heavy-water detector that significantly advanced understanding of solar neutrinos.
These facilities have enabled groundbreaking discoveries, contributing to the development of the Standard Model of particle physics and providing insights into astrophysical processes.
Unlocking Cosmic Mysteries
Neutrino detection plays a vital role in addressing some of the most profound questions about the universe’s origins, structure, and fate. By analyzing the behavior and sources of neutrinos, scientists have shed light on key cosmic phenomena.
Probing the Big Bang
Neutrinos are believed to have played a critical role in the early universe. Studying cosmic neutrino backgrounds—akin to the cosmic microwave background radiation—provides clues about the conditions that existed mere seconds after the Big Bang. These insights help refine cosmological models and the understanding of matter’s evolution.
Investigating Dark Matter and Dark Energy
Dark matter and dark energy collectively account for about 95% of the universe’s mass-energy content, yet their nature remains elusive. Neutrino studies have hinted at possible connections between these mysterious components and the behavior of subatomic particles. High-energy neutrinos from astrophysical sources provide unique opportunities to probe interactions that may reveal dark matter properties.
Understanding Stellar Evolution
Supernovae and neutron star collisions generate vast quantities of neutrinos, offering a detailed view of how stars evolve and die. The neutrinos from Supernova 1987A, for example, provided unprecedented information about core-collapse processes in massive stars.
Detecting Extraterrestrial Sources
Some of the most energetic neutrinos observed by the IceCube Observatory originate from distant cosmic phenomena, such as active galactic nuclei or gamma-ray bursts. These particles travel across billions of light-years, carrying information about regions of the universe otherwise inaccessible through electromagnetic observation.
Challenges in Neutrino Research
Although progress in neutrino science has been remarkable, significant challenges remain. For instance, precise measurement of neutrino masses, which are key to understanding their role in the universe, is still an open problem. Similarly, detecting neutrinos from specific sources, such as dark matter annihilation, requires new technologies and deeper underground facilities.
Overcoming Technological Barriers
Continuous development in detector sensitivity and resolution is essential for advancing the field. Next-generation observatories, such as the Hyper-Kamiokande in Japan and the KM3NeT in the Mediterranean, aim to expand detection capabilities significantly.
Collaboration and Interdisciplinary Approaches
Neutrino research benefits from close collaboration among physicists, astronomers, engineers, and computer scientists. Advanced computational techniques, such as machine learning, enhance data analysis and enable more efficient signal detection amidst background noise.
The Future of Neutrino Exploration
The coming decades promise exciting advancements in neutrino research. Some areas of focus include:
- Neutrino Astronomy: Using neutrinos to map and understand the universe’s most energetic and extreme phenomena.
- Neutrino Mass Hierarchy: Determining the exact ordering of neutrino masses, which has implications for the Standard Model and beyond.
- Cross-disciplinary Applications: Applying neutrino detection methods in fields such as geology and nuclear nonproliferation.
Emerging projects aim to address these goals while fostering global collaboration. As more countries invest in large-scale observatories, the field is poised to revolutionize our understanding of both microcosmic particles and the macrocosmic universe.
Conclusion
Neutrino detectors are at the forefront of exploring the secrets of the universe, offering unparalleled insight into cosmic events, subatomic interactions, and the fundamental nature of reality. As technology advances and international collaborations grow, the potential for groundbreaking discoveries continues to expand. By unlocking the mysteries of neutrinos, humanity gains a deeper understanding of the universe and its origins, shaping the future of science and our place within the cosmos.